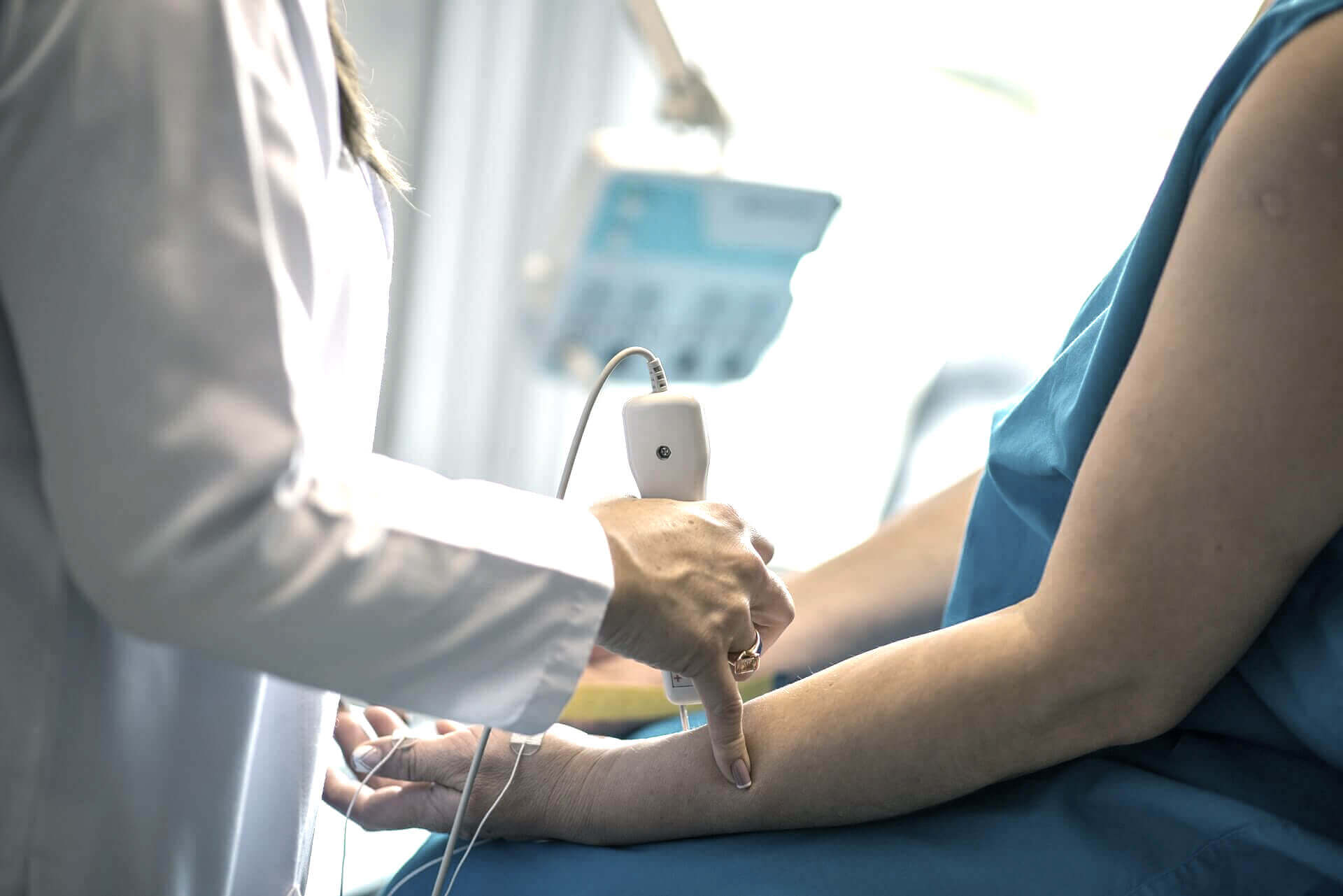
CK와 항암제의 병용에 따른
autophagy 활성화에 의한
항암치료
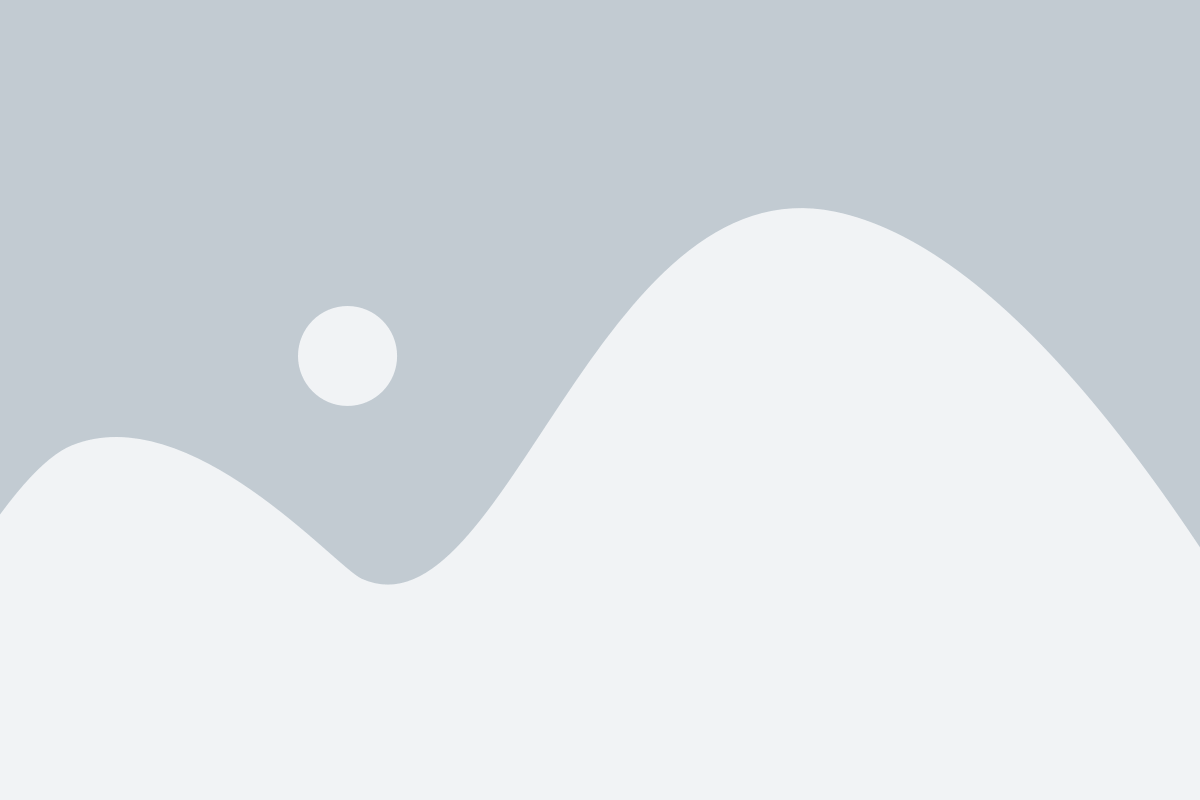
Ginsenoside compound K(CK)의 생산과 정제
인삼의 유효성분인 ginsenoside는 triterpenoid(protopanaxadiol, PPD)라는 탄소골격(carbon skeleton)에 당분자가 결합되어 있다. 탄소골격은 소수성이 높기 때문에 장 상피 세포막 투과가 용이하여 100% 체내 흡수율을 나타낸다. 그런데 탄소골격에 친수성 물질인 당분자가 결합되면 당분자 수에 비례하여 체내 흡수율이 낮아진다. 당 분자 4개가 결합된 진세노사이드 Rb1의 체내 흡수율은 절대 생체이용율(absolute bioavailability)로 1% 미만이다(Zhao et al., 2012). 그러나 당 분자 하나만이 결합된 진세노사이드 compound K(CK)는 사람 장내에서 100% 흡수됨이 밝혀졌다(Grès et al., 1998; Paek et al., 2006). 흡수 안된 진세노사이드들은 대부분 대변으로 배설된다.
당분자의 수와 종류만이 다른 Rb1, Rb2, Rc, Rd의 천연 ginsenoside들은 메주균(aspergillus niger)과 기타 식용세균의 발효에 의해서 CK로 전환된다. CK는 CK 발효물로부터 여러가지 물질 정제 방법으로 순도 30%에서 순도 99%이상인 결정체로 생산될 수 있다.
암조직에서의 염증반응
거식세포(macrophage)와 수지상세포(dendritic cell)의 표면에는 Toll-like receptor(TLR)가 결합되어 있다(Kawai and Akira, 2006). 이 수용체(receptor) 단백질은 초파리의 단백질인 toll과 유사한 구조를 가지고 있다. TLR에 결합할 수 있는 물질들은 TLR에 결합하여 이를 활성화함으로써 염증을 유발한다. 그러한 물질 중에는 병원균과 연관된 물질 PAMP(pathogen-associated molecular pattern)(Akira and Hemmi, 2003; Mahla et al., 2013)와 외부충격 또는 과격한 운동에 의해서 손상된 조직으로부터 유출된 물질 DAMP(damage-associated molecular pattern)가 있다(Hernandez et al., 2016; Roh and Sohn, 2018). 이상과 같은 PAMP와 DAMP는 선천성 면역세포(염증세포)를 통해서 염증을 유발한다.
PAMP중에서 대표적인 물질로서는 LPS(lipopolysaccharide)가 있다. LPS는 그람음성 세균의 세포막 구성성분의 일종으로서 지질에 당이 결합된 물질이다. 이 물질은 병원균 종류에 따라 지질 종류도 다양하고 당의 종류와 중합된 당의 숫자도 다르다(Miller et al., 2005; Klein and Raina, 2015). 그렇지만 사람의 선천성 면역계는 이 모두를 하나의 패턴으로 인식하여 염증을 유발한다.
TLR은 사람에서는 현재 10종류(Kawai and Akira, 2006)가 보고되었는데 이들이 인지하여 결합할 수 있는 물질의 종류는 다르다. 예를 들자면 LPS가 TLR4에 결합하면(Liu et al., 2008; Tong et al., 2020) TLR4의 세포내 영역(intracellular domain)은 활성화된다. 이어서 활성화된 세포내 영역에는 여러가지 접속분자(adaptor molecule)들이 결합하여 염증유발 싸이토카인(proinflammatory cytokine)의 발현을 유도할 수 있는 전사인자(transcription factor) NF-κB, AP-1, IRF3를 인산화한다. 이에 의해 활성화된 전사인자들은 세포핵으로 이행하여 이들 고유 promotor에 결합한 후에 여러가지 염증유발 싸이토카인 유전자의 발현을 유도한다.
NF-κB는 IL-1, IL-6, TNF-α, chemokines, cyclooxygenase-2(COX-2)등의 염증유발 싸이토카인의 발현을(Shih et al., 2015) 유도하고 AP-1은 IL-1(Kick et al., 1995)과 IL-6(Koyama et al., 1996), 이외의 여러가지 유전자의 발현을 유도한다. IRF3는 IFN-β등의 유전자 발현을 유도한다(Liu et al., 2008). IFN-β는 세포밖으로 분비된 후 염증세포 표면의 type Ⅰ interferon receptor에 결합하여 여러가지 염증유발 싸이토카인의 발현을 유도한다(Abe et al., 2008).
Chemokine이 분비되면 그 주위를 지나는 혈관 중의 면역세포들은 혈관벽을 투과한 후에 chemokine의 농도경사에 따라 이동하여 염증 발생 장소에 모여든다. 그리고 NF-κB는 cyclooxygenase-2(COX-2)를 발현시키어 부기와 통증을 유발한다.
Autophagy
생체는 에너지 생산 없이는 잠시도 생존할 수 없다. 따라서 생체는 끊임없이 ATP를 생산해야 한다. 이 때 사용한 산소의 대부분은 CO2 발생을 통해서 에너지 생산에 사용되지만 그 중 일부, 대략 3% 내외는 부산물로서 활성산소인 superoxide(O2-)로 전환된다 (Liu et al., 2002).
세포내에서 ATP를 생산하는 세포내 구조물(organelle)은 미토콘드리아다. 미토콘드리아를 구성하는 단백질들의 일부는 미토콘드리아 DNA에 의해 발현되고 일부는 세포핵 DNA에 의해서 발현된다(Candas and Li, 2014). 미토콘드리아 DNA는 세포핵 DNA보다 활성산소 발생장소와 밀접해 있기 때문에 DNA 변형율이 높다.
미토콘드리아는 활성산소로부터 DNA를 보호하기 위해서 Mn++ superoxide dismutase(Mn++SOD)를 가지고 있다(Candas and Li, 2014). 이 효소는 활성산소 superoxide(O2-)를 H2O2로 전환한다. H2O2는 미토콘드리아 막 지질의 aquaporin을 통해서(Bestetti et al., 2020) 세포질 내로 확산된 후 세포질 내의 catalase와 glutathione peroxidase(Guemouri et al., 1991; Michiels et al., 1994)에 의해서 물로 분해된다. 이러한 과정에서 미토콘드리아 DNA의 일부는 활성산소에 의한 변형을 피할 수 없다. 그 결과로 미토콘드리아는 ATP 생산기능을 상실한다. 세포는 기능 상실된 미토콘드리아를 새로운 미토콘드리아로 대체하여 ATP생산을 계속한다. 따라서 세포질 내에는 미토콘드리아가 복수(multicopy)로 존재해야 한다.
미토콘드리아는 세포내에서 큰 구조물에 해당한다. 따라서 기능 상실된 미토콘드리아를 분해하지 않은 상태로 미토콘드리아를 계속 생합성할 수 없다. 그래서 세포내에는 기능 상실된 미토콘드리아를 분해할 수 있는 기능을 갖춘 세포내 구조물이 존재한다. 이를 autophagosome(Mizushima, 2007)이라고 한다. Autophagosome은 세포막 지질로 내용물을 둘러싸고 있다. 그 내용물은 여러가지 단백질들의 복합체이다. 이와 같은 구조물은 단백질 변형에 의해 응집된 단백질들과 기능 상실된 여러가지 세포내 구조물을 막 안으로 끌어들인다. 그리고 lysosome과의 막 융합을 통해서 이들을 분해한다(Zhang et al., 2008). 이상과 같은 과정에서 미토콘드리아가 분해되면 미토콘드리아는 새로이 생합성된다(Nisoli et al., 2004; Ventura-Clapier and Veksler, 2008). 분해된 물질들은 세포내에서 재활용된다(Mizushima, 2007; Yang et al., 2011). 이와 같은 autophagosome의 처리 기능을 autophagy라고 한다. 그러나 세포내에 침투한 박테리아와 바이러스 등의 이물질을 분해하는 과정을 xenophagy라고 하는데 이것은 면역반응의 활성화(면역강화)로 이어진다. 그러나 여기서는 자세한 설명을 생략한다.
이상과 같이 autophagy는 세포 내의 항상성(cell homeostasis)을 유지하는 중요한 기능을 수행한다. 그러나 autophagy는 노화에 따라 쇠퇴하고 암을 비롯한 각종 염증성 질환에서는 억제된다.
영양 공급 부족으로 에너지 대사가 낮아지면 에너지 대사의 최상위에 있는 효소 AMPK가 활성화되어서(Laderoute et al. 2006) autophagosome 형성의 출발물질인 ULK1을 인산화한다(Kim et al., 2011; Egan et al., 2011). 그러나 영양공급이 충분해지면 mTOR가 ULK1을 위와 다른 부위에서 인산화함으로써 autophagosome 형성을 억제한다(Kim et al., 2011; Egan et al.; 2011). 인산화된 ULK1은 Atg 9 (autophagy-related protein 9) vesicle이 결합되어 있는 endoplasmic reticulum 일부를 분리한 후 분해대상 물질과 함께 복합체(complex)를 형성한다. 이와 같은 구조물을 ULK1 complex라고 한다(Karanasios et al., 2016).
Beclin-1은 autophagosome을 구성하는 단백질 일종으로서 여러 가지 Atg protein들과 복합체(complex)를 형성한다(Mizushima, 2010; Lin and Hurley, 2016). ULK1 complex가 Beclin-1을 인산화 하면 두가지 complex는 더 큰 complex를 이루고 세포막이 확대되어 이를 감싸면 autophagosome이 완성된다(Russell et al., 2013). Autophagosome의 구조는 효모에서 고등 진핵세포에 이르기까지 잘 보존되어 있다(Mizushima et al., 2002). 그런데 효모에서 이를 구성하는 단백질은 모두 31종이다(Mizushima, 2007).
Autophagy 억제에 의한 항암 효과
암조직은 발달 초기부터 저산소증(hypoxia)이 유지되고 있다(Zheng et al., 2015). 저산소증(Mazure and Pouysségur, 2010)에 의해 유발된 hypoxia-inducible factor 1(HIF-1)(Semenza et al., 2000)은 autophagy를 활성화한다(Bellot et al., 2009). 이에 따라 초기 암세포에서는 autophagy가 활성화된다.
Autophagy는 영양공급이 부족한 상태에서도 자가분해(self-digestion)로써 에너지를 생산할 수 있기 때문에 항암치료에서는 타격대상이 된다(Levy et al., 2017; Mowers et al., 2018). Autophagy 억제물질인 3-methyladenine(3-MA)은 대장암세포에서 5-fluorouracil(5-FU)에 의한 암세포의 세포자멸 효과를 증가시킨다(Li et al., 2009). 그리고 폐암세포(A549)에서 3-MA는 항암제 cisplatin과 paclitaxel에 의한 암세포의 세포자멸 효과를 증가시킨다(Liu et al., 2013). 그리고 실제로 임상시험에서도 autophagy 억제제가 사용되고 있다. Chude와 Amaravadi(2017)는 한 review article에서 앞으로 autophagy억제에 의한 항암 치료효과의 개선을 위해서는 autophagy에 특이한 더 강력한 억제제가 필요함을 주장하였다. 그리고 Xu등(2018)은 7개의 임상시험 결과의 분석을 통해서 autophagy 억제제 사용은 대조군에 비해 개선된 치료효과(better treatment response)를 보였다고 보고하였다.
Autophagy 활성화에 의한 항암 치료
이상의 주장과는 반대로 항암치료에서 autophagy를 활성화해야 할 필요성이 제기된다. 사람의 유방암, 난소암, 전립선암의 40~50%에서는 BECLIN1의 단일대립 유전자(monoallele)의 소실이 관찰된다(Levine and Kroemer, 2008). 이와 같은 사실은 정상적인 BECLIN1에 의한 autophagosome 형성은 암을 억제할 수 있음을 의미한다. 그리고 BECLIN1의 단일대립 유전자 한 개가 제거된 실험동물(heterozygote)에서는 autophagy가 억제되어(Qu et al., 2003) 자연적으로 림프구암, 폐암, 간암 등의 발생율이 높아진다.
암억제 유전자로 알려진 P53과 PTEN등은 autophagy가 활성화되어있는 상태에서는 암발생을 억제한다. 그러나 활성화 암유전자(activated oncogenes)인 PI3K, Akt, mTOR등은 autophagy를 억제함이 밝혀졌다(Mizushima et al., 2008).
암세포에서 autophagy를 활성화하면 암세포를 세포자멸로 유도할 수 있다. 소아의 급성 림프구성 백혈병(acute lymphoblastic leukemia, ALL)과 기타 림프구 암(lymphoid malignancies)에서 steroids(일명 glucocorticoids 또는 corticosteroids)는 60년 이상 사용되고 있다(Laane et al., 2009). 이 보고서에 따르면 스테로이드의 일종인 dexamethasone은 G1 phase cell cycle을 정지시키고 세포자멸을 유도함으로써 항암 효과를 나타낸다. Autophagy를 억제하기 위하여 beclin1의 발현을 억제하는 siRNA를 첨가한 ALL세포주 배양계에서는 siRNAs는 dexamethasone에 의한 세포자멸 효과를 추가적으로 증가시키지 않는다. 이것은 dexamethasone에 의한 ALL의 사멸은 autophagy 억제를 통해서 이루어지는 것이 아님을 의미한다. Dexamethasone은 ALL 세포주에서 promyelocytic leukemia protein(PML)의 발현을 유도한다. PML은 Akt와 complex을 이루면서 Akt의 탈 인산화를 유도한다. 이에 따라 autophagy가 활성화된다. 이어서 Bak과 Bax의 활성화에 의하여 세포자멸(apoptosis)억제물질인 Bcl-2와 Bcl-xL가 억제된다. 그 결과로 mitochondria의 세포자멸 경로가 활성화된다(Placzek et al., 2010). Dexamethasone은 이상과 같은 과정을 통해서 ALL을 포함한 림프구 암을 억제할 수 있다.
암 발생초기에는 HIF-1에 의해서 암세포에서는 autophagy가 활성화되지만 성장된 암조직에서는 이에 침투한 면역세포 TILs(tumor-infiltrating lymphocytes)이 많이 발견된다(Salgado et al., 2014; Stenzel et al., 2020)이것은 암조직에서 염증 반응이 활성화 되어있음 의미한다. 그리고 암환자의 혈중에서는 IL-1α, TNF-α, IL-6등의 염증유발 싸이토카인이 높게 측정된다(Mantovani et al., 2000). 이상과 같은 사실은 암은 전형적인 만성 염증성 질환임을 의미한다. 이와 같은 암 조직에서는 염증의 한가지 경로인 Akt/mTOR/NF-κB(Ahmad et al., 2013; Torrealba et al., 2020)가 활성화되어 있다. 이에 따라 암세포에서는 mTOR가 autophagy를 억제한다(Zoncu et al., 2011; Korkmaz et al., 2012). 이상과 같이 염증이 활성화된 암조직에서는 초기 암조직과는 달리 autophagy가 억제 되어있다. 이러한 상태에서 autophagy 촉진제의 사용이 얼마나 항암 치료효과에 도움이 될지 알아볼 필요가 있다.
CK는 AMPK 활성화(Kim et al., 2009)를 통해 mTOR를 억제한다(Shaw et al., 2004; Sugiyama et al., 2009). 그 결과로 CK는 autophagy를 활성화한다. CK는 이상과 같이 mTOR억제와 AMPK 활성화에 의한 ULK1의 인산화를 통해서 autophagy를 활성화한다. 따라서 CK는 암세포에서의 autophagy 활성화 효과는 뚜렷할 것으로 추정된다.
Autophagy는 JNKs의 활성화를 통해서 세포자멸을 유도할 수 있음이 Shimizu 등(2010)에 의해서 처음으로 밝혀졌다. JNKs는 세포자멸 유발(proapoptotic)단백질인 Bim과 Bmf를 활성화하고 이들은 이어서 Bax와 Bak를 활성화하여 세포자멸을 유도한다(Dhanasekaran and Reddy, 2008). CK는 이상과 같은 기능성으로써 고형암 세포주인 비 소세포 폐암(non-small cell lung cancer) 세포에서 암세포의 세포자멸을 유도한다(Li et al., 2019).
CK와 dexamethasone은 타격할 수 있는 암세포 종류에서 큰 차이를 보인다. Dexamethasone은 암 세포주에 고유한 단백질인 PML 발현을 통해 autophagy를 활성화함으로써 특정한 암세포주에서만 세포자멸을 유도하지만 CK는 암세포주와 무관하게 CK의 자체의 기능성으로써 autophagy를 활성화한다. 따라서 CK는 혈액암 뿐만아니라 고형암의 모든 암세포에서 세포자멸을 유도할 수 있을 것으로 판단된다.
현재 항암치료에서는 autophagy가 암세포의 생존을 촉진하거나 또는 억제하는 이중성을 보이는 것으로 보고되었다(Brech et al., 2009; Ávalos et al., 2014). 그리고 Levy 등(2017)은 암 초기단계의 조직에서 autophagy의 활성화는 암세포의 증식을 억제할 수 있으며 암이 진행된 조직에서는 autophagy의 활성화 또는 억제가 둘다 항암치료수단이 될 수 있음을 제안하였다. 그렇다면 항암치료에서 autophagy를 억제할 필요가 없다. Autophagy억제는 암세포에만 적용되지 않고 신체 모든 조직의 세포에도 적용된다. 신경 세포에서의 autophagy 억제는 알츠하이머 병 등의 신경퇴행성 질환을 유발할 수 있으며(Wong and Cuervo, 2010; Moloudizargari et al., 2017) 면역세포에서의 autophagy 억제는 면역억제를 통해서 특히 노인들을 감염성 질환에 취약하게 한다(Chen and White, 2011; Levin et al., 2011).
암세포를 포함한 모든 정상세포들은 저산소증에 노출되면 단백질 HIF-1(hypoxia-inducible factor 1)이 발현된다(Greijer and Wall, 2004; Semenza, 2000). HIF-1은 조직에 산소공급을 확대하기 위하여 혈관신생을 촉진하는 VEGF의 발현을 유도한다(Jung et al., 2005; Xu et al., 2005). 또한 HIF-1은 암세포에서 ATP 생산효율은 낮지만(1/16) 산소를 사용하지 않고 ATP를 생산할 수 있는 해당대사(glycolysis)를 활성화한다. 이를 Warburg 효과라고 한다(Bensinger and Christofk, 2012). 그리고 HIF-1은 미토콘드리아의 산소사용량을 감소시킨다(Papandreou et al., 2008) 따라서 암세포는 주로 glycolysis로 에너지를 생산하지 않을 수 없다. 이에 따라 암세포에서는 glycolysis를 통해서 포도당 한 분자로부터 ATP 두 분자와 lactate 두 분자를 생산한다. 암세포에서 유출된 두 분자의 lactate는 혈류에 따라 정상세포에 흡수된 후에 미토콘드리아의 대사를 통해 30분자의 ATP를 생산한다. 이상과 같이 에너지 생산 효율이 낮은 glycolysis로 암세포는 에너지를 생산하기 때문에 정상 세포와는 달리 포도당 수요가 대단히 높다. 그래서 암세포의 세포막에는 포도당 운반체 단백질 GLUT-1(Brown and Whal, 1993) 분자가 높은 밀도로 분포한다. 그런데 CK는 암세포에서 HIF-1과 GLUT-1을 억제함으로써 (Chen et al., 2019)암세포에서 에너지 생산을 효율적으로 감소시킬 수 있음이 밝혀졌다. 이에 따라 GLUT-1을 억제하는 항 GLUT-1 항체는 사람 암세포주들에서 성장정지(growth arrest)또는 세포자멸(apoptosis)을 유도하였고(Rastogi et al., 2007) GLUT-1의 발현을 억제하는 siRNA는 위암세포주 MKN45에서 Warburg효과를 역전시켰다(Zhang et al., 2015).
이상과 같이 항암치료에서 CK를 사용하면 GLUT-1의 억제를 통해서 암세포에서 에너지 생산을 크게 감소시킬 수 있지만 CK는 autophagy 활성화에 의해서는 자가분해를 통해서 에너지 생산을 증가시킬 수 있다. 그러나 autophagy에 의한 자가분해를 통한(Mizushima et al., 2008) 에너지 생산은 그 양이 일시적이고 제한적일 수 밖에 없다. 따라서 CK의 autophagy활성화에 따른 에너지 생산증가는 GLUT-1 억제에 의한 에너지 생산 감소에는 별 영향을 미치지 않을 것으로 보인다. 따라서 CK에 의한 autophagy활성화는 에너지 생산면에서는 항암치료에서 장애가 되지 않을 것으로 보인다.
세포 내에서 DNA가 손상을 받으면 암 억제유전자로 알려진 p53이 발현된 후에 여러 단계를 거쳐서 caspase를 활성화한다. 이에 의한 세포자멸로 암 발생이 억제된다. 그런데 p53은 유전자 변이가 빈번히 발생함에 따라 대부분의 암조직에서는 p53의 변이가 발견된다(Goh et al., 2011; Bykov et al., 2018). Rosenfeldt등(2013)은 사람 췌장선암(pancreatic ductal adenocarcinoma, PDAC)마우스 모델에서 p53과 autophagy관계를 연구하였다. p53이 정상인 상태에서 autophagy억제는 암발생을 감소시킨다. 그러나 p53이 결여된 상태에서 autophagy억제는 암 발생을 촉진한다. p53이 결여된 암세포에서 autophagy가 억제되면 포도당 흡수가 증가하고 동화대사(anabolic pathway)가 활성화된다. 이에 의해 암세포의 증식이 활발해진다. 따라서 p53의 변이 여부를 확인하지 않고 암환자에게 autophagy의 억제제 사용은 암을 악화시킬 수 있다.
스테로이드의 일종인 dexamethasone은 소아암 치료에서 autophagy 활성화를 통해 암세포를 억제한다. 그러나 스테로이드는 강력한 염증 억제제(Recio et al., 1995; Patel and Savjani, 2015)이지만 부작용으로서 골수억제(myelosuppression)(Mishell et al., 1982)를 동반한다. 골수세포들은 분화과정을 거쳐서 면역세포들과 그 밖의 혈구세포로 성장한다. 따라서 스테로이드는 이상과 같은 골수억제를 통해 면역억제(Stratta et al., 1988)뿐만 아니라 골밀도 감소(Ip et al., 1994; Peel et al., 1995), 소아의 발육지연(growth retardation)(Root et al., 1969) 등의 부작용도 나타난다. 그런데 스테로이드의 면역억제에 의한 세균감염은 생명에 위협이 될 수 있다(Hill et al., 2005). 그래서 스테로이드는 중단없이 계속 투여할 수 없다. 그래서 처음에는 고용량으로부터 점차 저용량으로 용량을 줄여 나가는(tapering)방법이 쓰이거나(Einaudi et al., 2008) 간헐적인 투여가 진행된다(Hill et al., 2005). 그렇지만 스테로이드가 소아암 치료에서 골수를 억제함에도 불구하고 치료약물로 사용될 수 있는 것은 소아는 골수억제로부터 회복이 빠르기 때문이다.
CK는 스테로이드의 부작용을 나타내지 않으며 autophagy 활성화를 통해서 암세포의 세포자멸을 유도한다. 따라서 소아 암환자에게 CK의 중단 없는 계속 투여는 항암치료 기간을 단축할 수 있으며 소아의 정상적인 발육을 유도할 수 있을 것으로 판단된다.
CK는 다른 약물의 도움 없이도 모든 암환자에서 단독투여에 따른 autophagy 활성화에 의해서 항암 효과를 나타낼 수 있다. 이때 CK에 의한 암세포의 증식 억제보다 암세포의 증식속도가 더 빠르면 암 억제 효과는 나타날 수 없다. 그렇지만 CK와 항암제와의 병용투여는 확실한 항암효과를 거둘 수 있을 것으로 보인다. CK는 autophagy 활성화를 통해서 암세포에서 세포자멸을 유도한다. 그런데 항암제는 분열하는 세포에 독성을 유발하는 물질이다. 따라서 두 가지 약물은 완전히 독립적인 기전으로 암세포의 세포자멸을 유도한다. 그 한 예로서 CK와 항암제 cisplatin을 유방암 세포주 MCF-7의 배양계에 첨가하면 암세포의 분열이 억제되며 암세포의 전이와 항암제 내성 발생과 관련된 epithelial mesenchymal transition(EMT)(Maier et al., 2010; Li et al., 2012)도 억제된다. 이상과 같은 두 가지 약물의 병용효과는 어느 한가지 약물의 효과보다도 높았다(Zhang and Li, 2016). 따라서 앞으로 임상시험에서도 항암제와 CK의 병용은 항암치료에서 상승효과를 나타낼 수 있을 것으로 예상된다.
항암제는 분열하는 세포에 독성을 유발하는 물질이다. 그런데 암세포는 세포분열이 빠르기 때문에 암조직은 항암제에 의한 타격이 크다. 그러나 신경세포를 제외한 각종 조직의 정상세포도 암세포보다는 느린 속도이지만 세포분열을 계속한다. 그 이유는 노화된 세포가 세포자멸로 소멸되면 이웃세포가 세포분열을 통해서 이를 복원하기 때문이다. 따라서 정상조직도 항암제에 의한 손상을 피할 길이 없다. 그러나 CK는 VEGF(vascular epithelial growth factor, 혈관 내피세포 성장인자)의 발현을 유도하여 손상된 조직을 시급히 복원할 수 있다. 이와 같은 논리는 암조직의 증식에도 적용된다. 따라서 현대의 항암치료에서는 VEGF억제제의 사용이 대세를 이루고 있다. 그렇지만 항암치료에서 VEGF 억제제 사용이 대세를 이루고 있다. 그렇지만 항암치료에서 VEGF억제제와 VEGF촉진제의 병용에 대한 이해득실을 따져 볼필요가 있다. 항암제와 VEGF억제제의 병용은 암조직의 위축을 촉진할 수 있다. 그러나 암조직은 신체전체조직의 극히 일부에 해당하고 정상조직은 신체전체조직이다. 따라서 장기간 항암치료를 받는 환자들 중에는 신체전체조직의 손상으로 극심한 체력손실을 겪는다. 이러한 경우에는 암환자는 항암치료를 계속 감당할 수 없기 때문에 항암치료 중단을 받아들일 수밖에 없다.
CK는 혈관 내피세포에서는 VEGF의 발현을 촉진하지만 암세포에서는 염증을 근본적으로 억제하여 암세포에서 분비되는 VEGF를 억제한다. 앞으로 항암치료에서 VEGF억제제의 병용과 VEGF촉진제의 병용의 이해득실에 대해서 별도로 설명할 예정이다.
mTOR는 serine/threonine kinase의 일종으로서 세포의 성장과 증식 그리고 여러 환경변화에 대응하는 생존에 관여하는 효소이다(Paquette et al., 2018). 그런데 초기 암조직과는 달리 진행된 암조직에서는 mTOR가 활성화되어 있고 이에 의해서 autophagy가 억제되어 있다. Teachey등(2008)은 mTOR억제제인 sirolimus와 항암제 methotrexate를 병용하여 ALL 세포주 배양계와 실험동물에서 ALL 억제에 대한 효과를 관찰하였던 바 위 두가지의 병용은 상승효과 (synergistic effect)가 있음을 보고하였다.
고농도 비타민 D3는 여러가지 암세포들을 억제함이 잘 알려져 있다(Abe et al., 1981; Guyton et al., 2003). 그런데 Wang 등(2008)은 고농도 비타민 D3가 Beclin1의 발현을 증가시키고 이것은 classⅢ PI3K와 결합하여 autophagy를 활성화함으로써 암세포를 억제한다고 밝혔다. 그리고 mTOR의 발현을 siRNA에 의해서 완전히 억제하면 Beclin1의 발현이 더욱 증가하고 이에 의한 autophagy 활성화는 비타민 D3의 leukemic cell의 억제를 더욱 강화하였다.
이상의 내용을 요약하면 CK는 암세포에서 mTOR억제를 통해 autophagy를 활성화하며 에너지대사의 최상위에 있는 AMPK를 활성화한다. AMPK는 autophagy기능을 수행하는 autophagosome형성의 출발물질인 ULK1을 인산화 하여 autophagy를 활성화한다. 그런데 Teachey등(2008)과 Wang등(2008)은 CK가 아닌 다른 물질로 mTOR를 억제하였고autophagy를 활성화하였다. 이러한 물질들이 항암 효과에 미치는 영향을 관찰하기 위하여 Teachey등은 mTOR 억제제와 항암제를 병용하였고 Wang등(2008)은 autophagy 활성화 물질과 항암제를 병용하였다. 이들은 이상과 같은 병용이 항암작용에서 상승효과를 나타냄을 보고하였다. 이상의 결과는 CK의 항암작용의 논리가 타당함을 증명해준다.
CK는 이상과 같이 항암치료에서 항암제의 부작용을 억제하고 항암효과에서 항암제와 상승효과(Synergistic Effect)를 보임으로써 항암치료의 새로운 패러다임(Paradigm)을 제공할 것으로 전망된다.
참고문헌
- Abe E., Miyaura C., Sakagami H., Takeda M., Konno K., Yamazaki T., Yoshiki S. and Suda T. (1981) Differentiation of mouse myeloid leukemia cells induced by 1 alpha,25-dihydroxyvitamin D3. Proc Natl Acad Sci. 78, 4990-4994.
- Abe M., Matsuda M., Kobayashi H., Miyata Y., Nakayama Y., Komuro R., Fukuhara A. and Shimomura I. (2008) Effects of statins on adipose tissue inflammation: their inhibitory effect on MyD88-independent IRF3/IFN-beta pathway in macrophages. Arterioscler Thromb Vasc Biol. 28, 871-877.
- Ahmad A., Biersack B., Li Y., Kong D., Bao B., Schobert R., Padhye S.B. and Sarkar F.H. (2013) Targeted regulation of PI3K/Akt/mTOR/NF-κB signaling by indole compounds and their derivatives: mechanistic details and biological implications for cancer therapy. Anticancer Agents Med Chem. 13, 1002-1013.
- Akira S. and Hemmi H. (2003) Recognition of pathogen-associated molecular patterns by TLR family. Immunol Lett. 85, 85-95.
- Ávalos Y., Canales J., Bravo-Sagua R., Criollo A., Lavandero S. and Quest A.F.G. (2014) Tumor suppression and promotion by autophagy. Biomed Re Int. 2014, 603980.
- Bellot G., Garcia-Medina R., Gounon P., Chiche J., Roux D., Pouysségur J. and Mazure N.M. (2009) Hypoxia-induced autophagy is mediated through hypoxia-inducible factor induction of BNIP3 and BNIP3L via their BH3 domains. Mol Cell Biol. 29, 2570-2581.
- Bensinger S.J. and Christofk H.R. (2012) New aspects of the Warburg effect in cancer cell biology. Semin Cell Dev Biol. 23, 352-361.
- Bestetti S., Galli M., Sorrentino I., Pinton P., Rimessi A., Sitia R. and Medraño-Fernandez I. (2020) Human aquaporin-11 guarantees efficient transport of H2O2 across the endoplasmic reticulum membrane. Redox Biology. 28, 101326.
- Brech A., Ahlquist T., Lothe R.A. and Stenmark H. (2009) Autophagy in tumour suppression and promotion. Molecular Oncology. 3, 366-375.
- Brown R.S. and Wahl R.L. (1993) Overexpression of Glut-1 glucose transporter in human breast cancer. An immunohistochemical study. Cancer. 72, 2979-2985.
- Bykov V.J.N., Eriksson S.E., Bianchi J. and Wiman K.G. (2018) Targeting mutant p53 for efficient cancer therapy. Nat Rev Cancer. 18, 89-102.
- Candas D. and Li J.J. (2014) MnSOD in oxidative stress response-potential regulation via mitochondrial protein influx. Antioxid Redox Signal. 20, 1599-1617.
- Chen H. and White E. (2011) Role of autophagy in cancer prevention. Cancer Prev Res. 4, 973-983.
- Chen H., Wu L., Li X., Zhu Y., Wang W., Xu C., Huang Z. and Du K. (2019) Ginsenoside compound K inhibits growth of lung cancer cells via HIF-1α-mediated glucose metabolism. Cell Mol Biol. 65, 48-52.
- Chude C.I. and Amaravadi R.K. (2017) Targeting Autophagy in Cancer: Update on Clinical Trials and Novel Inhibitors. Int J Mol Sci. 18, 1279.
- Dhanasekaran D.N. and Reddy E.P. (2008) JNK Signaling in Apoptosis. Oncogene. 27, 6245-6251.
- Egan D.F., Kim J., Shaw R.J. and Guan K. (2011) The autophagy initiating kinase ULK1 is regulated via opposing phosphorylation by AMPK and mTOR. Autophagy. 7, 645-646.
- Einaudi S., Bertorello N., Masera N., Farinasso L., Barisone E., Rizzari C., Corrias A., Villa A., Riva F., Saracco P. and Pastore G. (2008) Adrenal axis function after high-dose steroid therapy for childhood acute lymphoblastic leukemia. Pediatr Blood Cancer. 50, 537-541.
- Goh A.M., Coffill C.R. and Lane D.P. (2011) The role of mutant p53 in human cancer. J Pathol. 223, 116-126.
- Greijer A.E. and van der Wall E. (2004) The role of hypoxia inducible factor 1 (HIF-1) in hypoxia induced apoptosis. J Clin Pathol, 57, 1009-1014.
- Grès M.C., Julian B., Bourrié M., Meunier V., Roques C., Berger M., Boulenc X., Berger Y. and Fabre G. (1998) Correlation between oral drug absorption in humans, and apparent drug permeability in TC-7 cells, a human epithelial intestinal cell line: comparison with the parental Caco-2 cell line. Pharm Res. 15, 726-733.
- Guemouri L., Artur Y., Herbeth B., Jeandel C., Cuny G. and Siest G. (1991) Biological variability of superoxide dismutase, glutathione peroxidase, and catalase in blood. Clinical Chemistry. 37, 1932-1937.
- Guyton K.Z., Kensler T.W. and Posner G.H. (2003) Vitamin D and vitamin D analogs as cancer chemopreventive agents. Nutr Rev. 61, 227-238.
- Hernandez C., Huebener P. and Schwabe R.F. (2016) Damage-associated molecular patterns in cancer: a double-edged sword. Oncogene. 35, 5931-5941.
- Hill G., Chauvenet A.R., Lovato J. and McLean T.W. (2005) Recent steroid therapy increases severity of varicella infections in children with acute lymphoblastic leukemia. Pediatrics. 116, e525-529.
- Ip M., Lam K., Yam L. Kung A. and Ng M. (1994) Decreased Bone Mineral Density in Premenopausal Asthma Patients Receiving Long-Term Inhaled Steroids. Chest. 105, 1722-1727.
- Jung J.E., Lee H., Cho I., Chung D.H., Yoon S., Yang Y.M., Lee J.W., Choi S., Park J., Ye S. and Chung M. (2005) STAT3 is a potential modulator of
HIF-1-mediated VEGF expression in human renal carcinoma cells. The FASEB Journal. 19, 1296-1298. - Karanasios E., Walker S.A., Okkenhaug H., Manifava M., Hummel E., Zimmermann H., Ahmed Q., Domart M., Collinson L. and Ktistakis N.T. (2016) Autophagy initiation by ULK complex assembly on ER tubulovesicular regions marked by ATG9 vesicles. Nat Commun. 7, 12420.
- Kawai T. and Akira S. (2006) TLR signaling. Cell Death Differ. 13, 816-825.
- Kick G., Messer G., Goetz A., Plewig G. and Kind P. (1995) Photodynamic therapy induces expression of interleukin 6 by activation of AP-1 but not NF-kappa B DNA binding. Cancer Res. 55, 2373-2279.
- Kim D.Y., Yuan H.D., Chung I.K. and Chung S.H. (2009) Compound K, intestinal metabolite of ginsenoside, attenuates hepatic lipid accumulation via AMPK activation in human hepatoma cells. J Agric Food Chem. 57, 1532-1537.
- Kim J., Kundu M., Viollet B. and Guan K. (2011) AMPK and mTOR regulate autophagy through direct phosphorylation of Ulk1. Nat Cell Biol. 13, 132-141.
- Klein G. and Raina S. (2015) Regulated Control of the Assembly and Diversity of LPS by Noncoding sRNAs. Biomed Res Int. 2015, 153561.
- Korkmaz G., le Sage C., Tekirdag K.A., Agami R. and Gozuacik D. (2012) miR-376b controls starvation and mTOR inhibition-related autophagy by targeting ATG4C and BECN1. Autophagy. 8, 165-176.
Koyama Y., Tanaka Y., Saito K., Abe M., Nakatsuka K., Morimoto I., Auron P.E. and Eto S. (1996) Cross-linking of intercellular adhesion molecule 1 (CD54) induces AP-1 activation and IL-1beta transcription. J Immunol. 157, 5097-5103. - Laane E., Tamm K.P., Buentke E., Ito K., Khahariza P., Oscarsson J., Corcoran M., Björklund A., Hultenby K., Lundin J., Heyman M., Söderhäll S., Mazur J., Porwit A., Pandolfi P.P., Zhivotovsky B., Panaretakis T. and Grandér D. (2009) Cell death induced by dexamethasone in lymphoid leukemia is mediated through initiation of autophagy. Cell Death & Differentiation. 16, 1018-1029.
- Laderoute K.R., Amin K., Calaoagan J.M., Knapp M., Le T., Orduna J., Foretz M. and Viollet B. (2006) 5′-AMP-activated protein kinase (AMPK) is induced by low-oxygen and glucose deprivation conditions found in solid-tumor microenvironments. Mol Cell Biol. 26, 5336-5347.
- Levine B. and Kroemer G. (2008) Autophagy in the pathogenesis of disease. Cell. 132, 27-42.
- Levine B., Mizushima N. and Virgin H.W. (2011) Autophagy in immunity and inflammation. Nature. 469, 323-335.
- Levy J.M.M., Towers C.G. and Thorburn A. (2017) Targeting autophagy in cancer. Nat Rev Cancer. 17, 528-542.
- Li C., Dong Y., Wang L., Xu G., Yang Q., Tang X., Qiao Y. and Cong Z. (2019) Ginsenoside metabolite compound K induces apoptosis and autophagy in non-small cell lung cancer cells via AMPK-mTOR and JNK pathways. Biochem Cell Biol. 97, 406-414.
- Li C., Xia W., Huo L., Lim S.O., Wu Y., Hsu J.L., Chao C, Yamaguchi H., Yang N.K., Ding Q., Wang Y., Lai Y., LaBaff A.M., Wu T.J., Lin B.R., Yang M.H., Hortobagyi G.N. and Hung M. (2012) Epithelial-mesenchymal transition induced by TNF-α requires NF-κB-mediated transcriptional upregulation of Twist1. Cancer Res. 72, 1290-1300.
- Li J., Hou N., Faried A., Tsutsumi S., Takeuchi T. and Kuwano H. (2009) Inhibition of autophagy by 3-MA enhances the effect of 5-FU-induced apoptosis in colon cancer cells. Ann Surg Oncol. 16, 761-771.
- Lin M.G. and Hurley J.H. (2016) Structure and function of the ULK1 complex in autophagy. Curr Opin Cell Biol. 39, 61-68.
- Liu F., Liu D., Yang Y. and Zhao S. (2013) Effect of autophagy inhibition on chemotherapy-induced apoptosis in A549 lung cancer cells. Oncol Lett. 5, 1261-1265.
- Liu X., Yao M., Li N., Wang C., Zheng Y. and Cao X. (2008) CaMKII promotes TLR-triggered proinflammatory cytokine and type I interferon production by directly binding and activating TAK1 and IRF3 in macrophages. Blood. 112, 4961-4970.
- Liu Y., Fiskum G. and Schubert D. (2002) Generation of reactive oxygen species by the mitochondrial electron transport chain. J Neurochem. 80, 780-787.
Mahla R.S., Reddy M.C., Prasad D.V.R. and Kumar H. (2013) Sweeten PAMPs: Role of Sugar Complexed PAMPs in Innate Immunity and Vaccine Biology. Front Immunol. 4, 248. - Maier H.J., Schmidt-Strassburger U., Huber M.A., Wiedemann E.M., Beug H. and Wirth T. (2010) NF-kappaB promotes epithelial-mesenchymal transition, migration and invasion of pancreatic carcinoma cells. Cancer Lett. 295, 214-228.
- Mantovani G., Macciò A., Mura L., Massa E., Mudu M.C., Mulas C., Lusso M.R., Madeddu C. and Dessì A. (2000) Serum levels of leptin and proinflammatory cytokines in patients with advanced-stage cancer at different sites. J Mol Med. 78. 554-561.
- Mazure N.M. and Pouysségur J. (2010) Hypoxia-induced autophagy: cell death or cell survival? Curr Opin Cell Biol. 22, 177-180.
- Michiels C., Raes M., Toussaint O. and Remacle J. (1994) Importance of Se-glutathione peroxidase, catalase, and Cu/Zn-SOD for cell survival against oxidative stress. Free Radic Biol Med. 17, 235-248.
- Miller S.I., Ernst R.K. and Bader M.W. (2005) LPS, TLR4 and infectious disease diversity. Nat Rev Microbiol. 3, 36-46.
- Mishell R.I., Lee D.A., Grabstein K.H. and Lachman L.B. (1982) Prevention of the in vitro myelosuppressive effects of glucocorticosteroids by interleukin 1 (IL 1). J Immunol. 128, 1614-1619.
- Mizushima N. (2007) Autophagy: process and function. Genes Dev. 21, 2861-2873.
- Mizushima N. (2010) The role of the Atg1/ULK1 complex in autophagy regulation. Curr Opin Cell Biol. 22, 132-139.
- Mizushima N., Levine B., Cuervo A.M. and Klionsky D.J. (2008) Autophagy fights disease through cellular self-digestion. Nature. 451, 1069-1075.
- Mizushima N., Ohsumi Y. and Yoshimori T. (2002) Autophagosome formation in mammalian cells. Cell Struct Funct. 27, 421-429.
- Moloudizargari M., Asghari M.H., Ghobadi E., Fallah M., Rasouli S. and Abdollahi M. (2017) Autophagy, its mechanisms and regulation: Implications in neurodegenerative diseases. Ageing Res Rev. 40, 64-74.
- Mowers E.E., Sharifi M.N. and Macleod K.F. (2018) Functions of autophagy in the tumor microenvironment and cancer metastasis. FEBS J. 285,1751-1766.
- Nisoli E., Falcone S., Tonello C., Cozzi V., Palomba L., Fiorani M., Pisconti A., Brunelli S., Cardile A., Francolini M., Cantoni O., Carruba M.O., Moncada S. and Clementi E. (2004) Mitochondrial biogenesis by NO yields functionally active mitochondria in mammals. Proc Natl Acad Sci. 101, 16507-16512.
- Paek I.B., Moon Y., Kim O., Ji H.Y., Kim S.A., Sohn D.H., Kim J.B. and Lee H.S. (2006) Pharmacokinetics of a ginseng saponin metabolite compound K in rats. Biopharm Drug Dispos. 27, 39-45.
- Papandreou I., Lim A.L., Laderoute K. and Denko N.C. (2008) Hypoxia signals autophagy in tumor cells via AMPK activity, independent of HIF-1, BNIP3, and BNIP3L. Cell Death & Differentiation. 15, 1572-1581.
- Paquette M., El-Houjeiri L. and Pause A. (2018) mTOR Pathways in Cancer and Autophagy. Cancers. 10, 18.
- Patel S.S. and Savjani J.K. (2015) Systematic review of plant steroids as potential antiinflammatory agents: Current status and future perspectives. The Journal of Phytopharmacology. 4, 121-125
- Peel N.F., Moore D.J., Barrington N.A., Bax D.E. and Eastel R. (1995) Risk of vertebral fracture and relationship to bone mineral density in steroid treated rheumatoid arthritis. Ann Rheum Dis. 54, 801–806.
- Placzek W.J., Wei J., Kitada S., Zhai D., Reed J.C. and Pellecchia M. (2010) A survey of the anti-apoptotic Bcl-2 subfamily expression in cancer types provides a platform to predict the efficacy of Bcl-2 antagonists in cancer therapy. Cell Death Dis. 1, e40.
- Qu X., Yu J., Bhagat G., Furuya N., Hibshoosh H., Troxel A., Rosen J., Eskelinen E., Mizushima N. Ohsumi Y., Cattoretti G. and Levine B. (2003) Promotion of tumorigenesis by heterozygous disruption of the beclin 1 autophagy gene. J Clin Invest. 112, 1809-1820.
- Rastogi S., Banerjee S., Chellappan S. and Simon G.R. (2007) Glut-1 antibodies induce growth arrest and apoptosis in human cancer cell lines. Cancer Lett. 257, 244-251.
- Recio M.C., Giner R.M., Máñez S., Gueho J., Julien H.R., Hostettmann K. and Ríos J.L. (1995) Investigations on the steroidal anti-inflammatory activity of triterpenoids from Diospyros leucomelas. Planta Med. 61, 9-12.
- Roh J.S. and Sohn D.H. (2018) Damage-Associated Molecular Patterns in Inflammatory Diseases. Immune Netw. 18, e27.
- Root A.W., Bongiovanni A.M. and Eberlein W.R. (1969) Studies of the secretion and metabolic effects of human growth hormone in children with glucocorticoid-induced growth retardation. The Journal of Pediatrics. 75, 826-832.
- Rosenfeldt M.T., O’Prey J., Morton J.P., Nixon C., MacKay G., Mrowinska A., Au A., Rai T.S., Zheng L., Ridgway R., Adams P.D., Anderson K.I., Gottlieb E., Sansom O.J. and Ryan K.M. (2013) p53 status determines the role of autophagy in pancreatic tumour development. Nature. 504, 296-300.
- Russell R.C., Tian Y., Yuan H., Park H.W., Chang Y., Kim J., Kim H., Neufeld T.P., Dillin A. and Guan K. (2013) ULK1 induces autophagy by phosphorylating Beclin-1 and activating VPS34 lipid kinase. Nature Cell Biology. 15, 741-750.
- Salgado R., Denkert C., Demaria S., Sirtaine N., Klauschen F., Pruneri G., Wienert S., Van den Eynden G., Baehner F.L., Penault-Llorca F., Perez E.A., Thompson E.A., Symmans W.F., Richardson A.L., Brock J., Criscitiello C., Bailey H., Ignatiadis M., Floris G., Sparano J., Kos Z., Nielsen T., Rimm D.L., Allison K.H., Reis-Filho J.S., Loibl S., Sotiriou C., Viale G., Badve S., Adams S., Willard-Gallo K., Loi S. and International TILs Working Group. (2014) The evaluation of tumor-infiltrating lymphocytes (TILs) in breast cancer: recommendations by an International TILs Working Group 2014. Ann Oncol. 26, 259-271.
- Semenza G.L., Agani F., Feldser D., Iyer N., Kotch L., Laughner E. and Yu A. (2000) Hypoxia, HIF-1, and the pathophysiology of common human diseases. Adv Exp Med Biol. 475, 123-130.
- Shaw R.J., Bardeesy N., Manning B.D., Lopez L., Kosmatka M., DePinho R.A. and Cantley L.C. (2004) The LKB1 tumor suppressor negatively regulates mTOR signaling. Cancer Cell. 6, 91-99.
- Shih R., Wang C. and Yang C. (2015) NF-kappaB Signaling Pathways in Neurological Inflammation: A Mini Review. Front Mol Neurosci. 8, 77.
- Shimizu S., Konishi A., Nishida Y., Mizuta T., Nishina H., Yamamoto A. and Tsujimoto Y. (2010) Involvement of JNK in the regulation of autophagic cell death. Oncogene. 29, 2070-2082.
- Stenzel P.J., Schindeldecker M., Tagscherer K.E., Foersch S., Herpel E., Hohenfellner M., Hatiboglu G., Alt J., Thomas C., Haferkamp A., Roth W. and Macher-Goeppinger S. (2020) Prognostic and Predictive Value of Tumor-infiltrating Leukocytes and of Immune Checkpoint Molecules PD1 and PDL1 in Clear Cell Renal Cell Carcinoma. Transl Oncol. 13, 336-345.
- Stratta R.J., Armbrust M.J., Oh C.S., Pirsch J.D., Kalayoglu M., Sollinger H.W. and Belzer F.O. (1988) Withdrawal of Steroid Immunosuppression in Renal Transplant Recipients. Transplantation. 45, 323-328.
- Sugiyama M., Takahashi H., Hosono K., Endo H., Kato S., Yoneda K., Nozaki Y., Fujita K., Yoneda M., Wada K., Nakagama H. and Nakajima A. (2009) Adiponectin inhibits colorectal cancer cell growth through the AMPK/mTOR pathway. Int J Oncol. 34, 339-344.
- Teachey D.T., Sheen C., Hall J., Ryan T., Brown V.I., Fish J., Reid G.S.D, Seif A.E., Norris R., Chang Y.J., Carroll M. and Grupp S.A. (2008) mTOR inhibitors are synergistic with methotrexate: an effective combination to treat acute lymphoblastic leukemia. Blood. 112, 2020-2023.
- Tong W., Chen X., Song X., Chen Y., Jia R., Zou Y., Li L., Yin L., He C., Liang X., Ye G., Lv C., Lin J. and Yin Z. (2020) Resveratrol inhibits LPS-induced inflammation through suppressing the signaling cascades of TLR4-NF-κB/MAPKs/IRF3. Exp Ther Med. 19, 1824-1834.
- Torrealba N., Vera R., Fraile B., Martínez-Onsurbe P., Paniagua R. and Royuela M. (2020) TGF-β/PI3K/AKT/mTOR/NF-kB pathway. Clinicopathological features in prostate cancer. Aging Male. 23, 801-811.
- Ventura-Clapier R., Garnier A. and Veksler V. (2008) Transcriptional control of mitochondrial biogenesis: the central role of PGC-1alpha. Cardiovasc Res. 79, 208-217.
- Wang J., Lian H., Zhao Y., Kauss M.A. and Spindel S. (2008) Vitamin D3 induces autophagy of human myeloid leukemia cells. J Biol Chem. 283, 25596-25605.
- Wong E. and Cuervo A.M. (2010) Autophagy gone awry in neurodegenerative diseases. Nat Neurosci. 13, 805-811.
- Xu Q., Briggs J., Park S., Niu G., Kortylewski M., Zhang S., Gritsko T., Turkson J., Kay H., Semenza G.L., Cheng J.Q., Jove R. and Yu H. (2005) Targeting Stat3 blocks both HIF-1 and VEGF expression induced by multiple oncogenic growth signaling pathways. Oncogene. 24, 5552-5560.
- Xu R., Ji Z., Xu C. and Zhu J. (2018) The clinical value of using chloroquine or hydroxychloroquine as autophagy inhibitors in the treatment of cancers. Medicine. 97, e12912.
- Yang Z.J., Chee C.E., Huang S. and Sinicrope F.A. (2011) The role of autophagy in cancer: therapeutic implications. Mol Cancer Ther. 10, 1533-1541.
- Zhang H., Bosch-Marce M., Shimoda L.A., Tan Y.S., Baek J.H., Wesley J.B., Gonzalez F.J. and Semenza G.L. (2008) Mitochondrial Autophagy Is an HIF-1-dependent Adaptive Metabolic Response to Hypoxia. J Biol Chem. 283, 10892-10903.
- Zhang K. and Li Y. (2016) Effects of ginsenoside compound K combined with cisplatin on the proliferation, apoptosis and epithelial mesenchymal transition in MCF-7 cells of human breast cancer. Pharm Biol. 54, 561-568.
- Zhang T., Zhao Y., Tong Z. and Guan Y. (2015) Inhibition of glucose-transporter 1 (GLUT-1) expression reversed Warburg effect in gastric cancer cell MKN45. Int J Clin Exp Med. 15, 2423-2428.
- Zhao J., Su C., Yang C., Liu M., Tang L., Su W. and Liu Z. (2012) Determination of ginsenosides Rb1, Rb2, and Rb3 in rat plasma by a rapid and sensitive liquid chromatography tandem mass spectrometry method: Application in a pharmacokinetic study. J Pharm Biomed Anal. 64-65, 94-97.
- Zheng X., Wang X., Mao H., Wu W., Liu B. and Jiang X. (2015) Hypoxia-specific ultrasensitive detection of tumours and cancer cells in vivo. Nature Communications. 6, 5834.
- Zoncu R., Efeyan A. and Sabatini D.M. (2011) mTOR: from growth signal integration to cancer, diabetes and ageing. Nature Reviews Molecular Cell Biology. 12, 21-35.
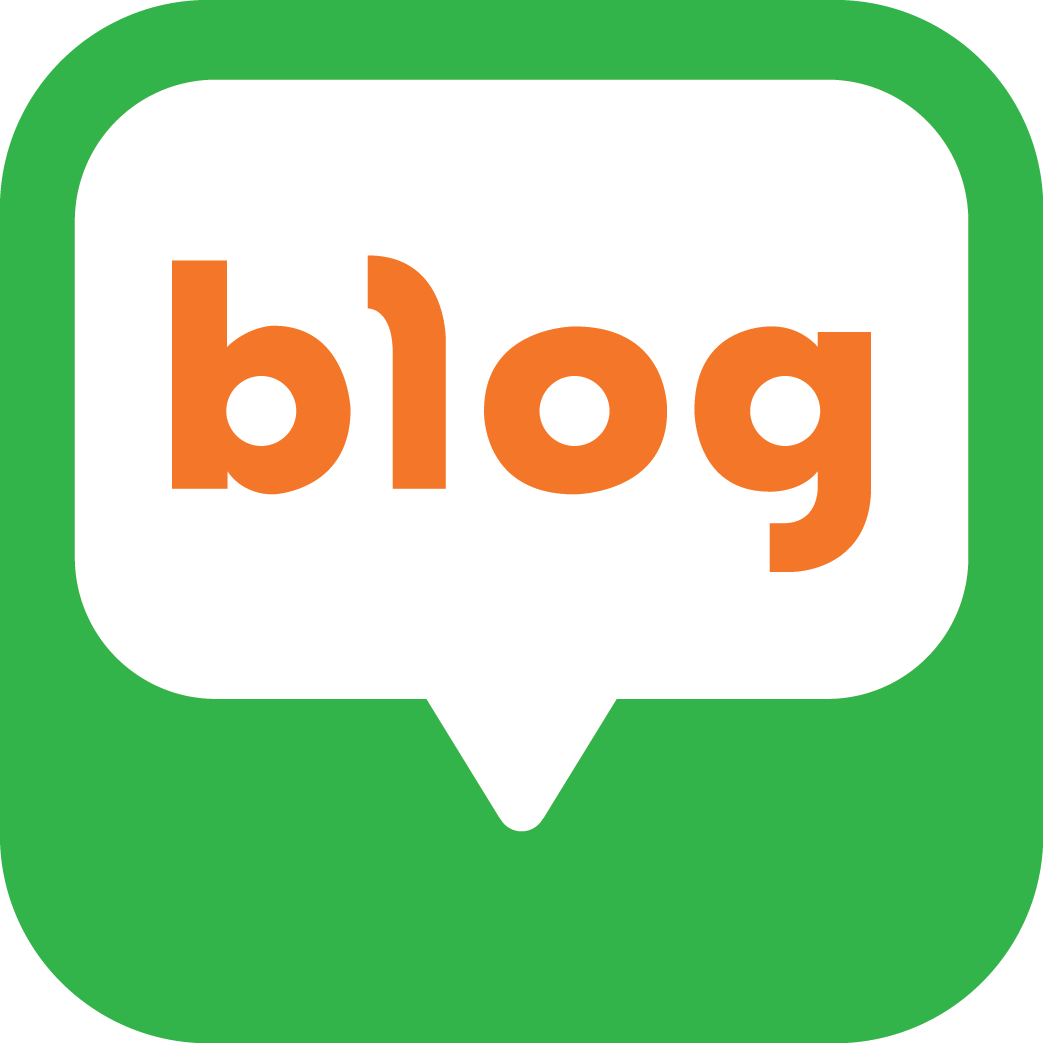
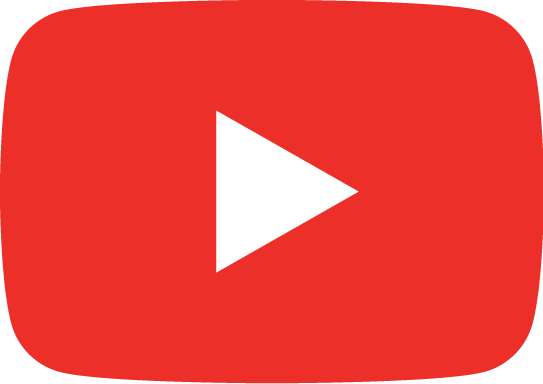
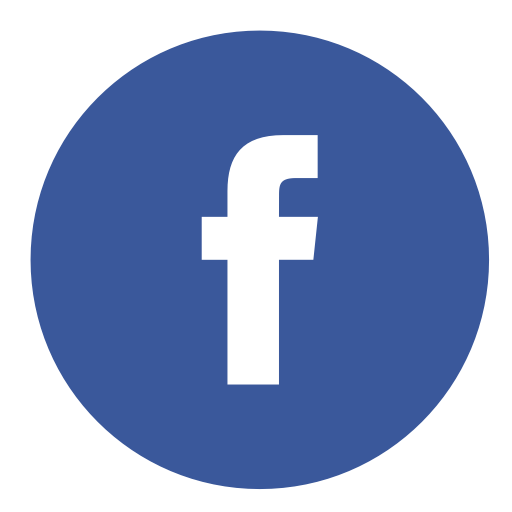
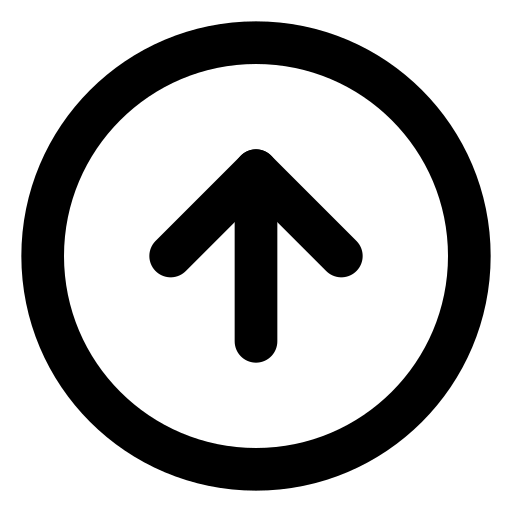